Martensitic Stainless Steel for Knife Applications
A high hardness level, a fine array of uniformly distributed primary alloy carbides, and an adequate matrix chromium content are the three most desired properties required to produce a knife with optimum properties. Ideally, a martensitic stainless steel grade for knife applications should, therefore, satisfy the following two fundamental requirements:
The carbon content of the austenitic matrix has to be around 0.6 wt. pct. or higher in order to achieve the hardness of 63-64 HRC. The chromium content of the austenitic matrix has to be at least 12 wt. pct. in order to ensure corrosion resistance. It should be said, however, that a part of matrix chromium can be replaced with molybdenum with little or no negative consequences for corrosion resistance.
Isothermal sections of the Fe-Cr-C ternary phase diagram are a good starting point when it comes to understanding the various trade-offs between the austenitization temperature selected for heat treatment and the resulting chemical composition of the austenitic matrix. The composition plane for the Fe-Cr-C ternary phase diagram at 1000°C (1832°F) is shown on Figure 1. The carbon content is plotted along the horizontal axis and the chromium content along the vertical axis of the composition plane.
The isothermal section in Figure 1 shows the content of chromium and carbon in the various phases of Fe-Cr-C alloys that can exist at 1000°C (1832°F). The area labeled γ (the Greek letter gamma) represents austenite. If the composition of an Fe-Cr-C alloy is plotted on Figure 1 and it falls inside the γ area, the microstructure of that alloy will consist of austenite only, i.e., no carbides will be present at 1000°C (1832°F).
Consider now one of the most basic martensitic stainless steel grades such as AISI 440C (approximately 17 wt. pct. chromium and 1.075 wt. pct. carbon), plotted on the composition plane of Figure 1. Its chemical composition falls inside of the region labeled γ + M7C3. This means that if AISI 440C is heated to 1000°C (1832°F) its microstructure will consist of austenite and chromium-rich M7C3 primary carbides. Upon quenching the martensite formed from the austenite will contain chromium-rich M7C3 primary carbides dispersed within it.
It is worth noting that on Figure 1 the right-hand boundary of the austenite region is labeled "Carbon Saturation Line". This line is important as it tells us the maximum amount of carbon that austenite can dissolve within itself — addition of more carbon would precipitate carbides.
The further the alloy composition lies to the right of the saturation line, the larger the volume fraction of chromium-rich M7C3 primary carbides it will contain. The presence of chromium-rich M7C3 primary carbides renders the austenite depleted in both chromium and carbon relative to the overall chemical composition of the alloy.
Figure 1 can be used to determine the chemical composition of the austenite in an alloy such as AISI 440C. The austenite composition for AISI 440C at 1000°C (1832°F) is found at the point where the tie line drawn through AISI 440C intersects the carbon saturation line. It is worth noting that even though AISI 440C alloy contains 1.075 percent of carbon and 17 percent of chromium overall, the austenite that forms at 1000°C (1832°F) contains only around 0.3 percent of carbon and 11.7 percent of chromium (see Figure 1). The martensite that forms upon quenching has the same chemical composition as the austenite. The carbon and chromium contents of the martensite have, in turn, the effect on its hardness and corrosion resistance, respectively. Thus, AISI 440C martensitic stainless steel, when hardened from 1000°C (1832°F), does not satisfy the two requirements stated above (the carbon and chromium content of the matrix of at least 0.6 and 12 percent, respectively).
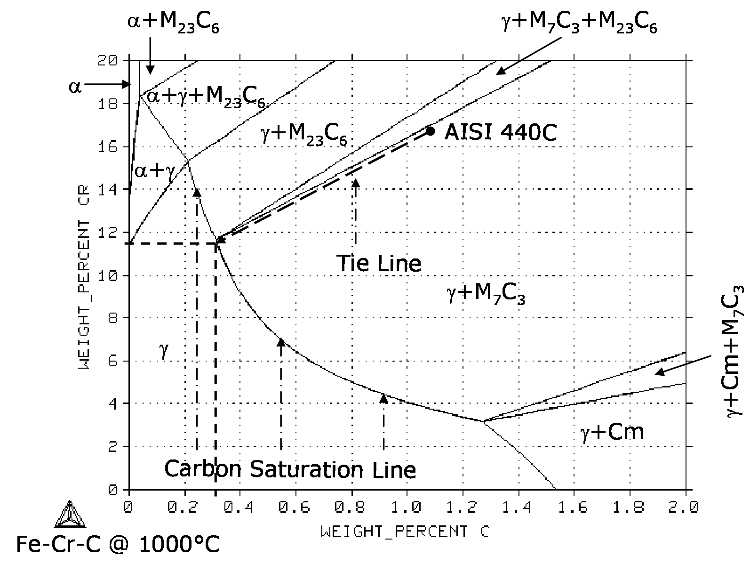
Figure 1. Isothermal section of Fe-Cr-C ternary phase diagram at 1000°C (1832°F) calculated with Thermo-Calc coupled with TCFE2000 thermodynamic database.
To demonstrate the effect of increasing the austenitization temperature on the volume fraction of primary carbides and the chemical composition of the austenite, consider the change in the position of the carbon saturation line in Figure 2.
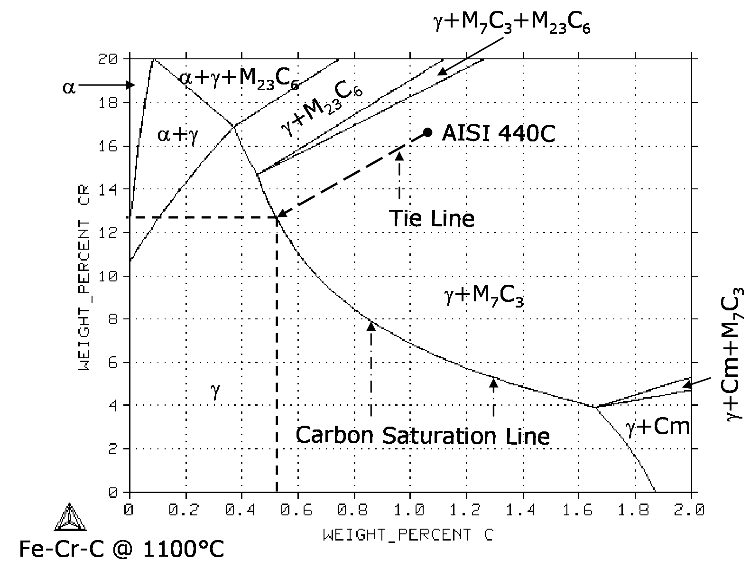
Figure 2. Isothermal section of Fe-Cr-C ternary phase diagram at 1100°C (2012°F) calculated with Thermo-Calc coupled with TCFE2000 thermodynamic database.
When the austenitization temperature is increased from 1000°C (1832°F) to 1100°C (2012°F), the content of carbon and chromium in the austenitic matrix is increased from 0.3 % C / 11.7 % Cr to 0.5 % C / 13.2 % Cr. The volume fraction of chromium-rich M7C3 primary carbides is smaller at 1100°C (2012°F) than at 1000°C (1832°F), as graphically demonstrated by the length of the tie line in Figures 1 and 2.
The Second Edition of Heat Treater's Guide — Practice and Procedures for Irons and Steels (published by ASM International in 1995) recommends that AISI 440C be austenitized at 1010°C-1065°C (1850°F-1949°F). For maximum corrosion resistance and strength, the Guide recommends the upper end of the austenitization range. Such a recommendation is not surprising. The above given phase diagrams demonstrate that the higher austenitization temperatures will increase both the carbon and chromium contents of the austenitic matrix.
It is worth noting that the matrix of AISI 440C martensitic stainless steel does not simultaneously satisfy the 0.6 % C and 12 % Cr requirements, even at 1100°C (2012°F). Not surprisingly, AISI 440C is typically hardened to just 58-60 HRC.
Finally, the Fe-Cr-C ternary phase diagrams explain — at least in part — why some commercial martensitic stainless steel tube grades, such as Sandvik 12C27 (Fe-0.6C-13.5Cr) and Uddeholm AEB-L Fe-0.65C-12.8Cr, are in general considered to be well optimized for stailess knives.
Austenitic | Martensitic | Ferritic | Duplex | Super Duplex | Superaustenitic | Superferritic | Precipitation Hardening
Martensitic and Precipitation Hardening Steel Comparison Table
|