Selection 316L Stainless Steel for Semiconductor Gas Filter
The continued increase in semiconductor device sophistication, and resultant decrease in line widths, require cleaner components throughout process gas delivery systems. Properly designed high purity gas filter assemblies are transparent to homogeneous constituents in the gas stream, i.e., to remove particulate contamination with no other effect on the inlet gas purity. In order to accomplish this goal, the assemblies used to house the filter devices are most often constructed of smooth, clean and electropolished 316L stainless steel.
“Smooth and clean” is a relative term, requiring more explicit definition. Specification requirements are established whenever performance limits for filter assemblies are clearly understood and measurable. For example, moisture contribution and surface roughness are reasonably well defined. However, these criteria are based on measurements limited by the sensitivity of the best available instrumentation. In order to meet these criteria, the “best” available raw materials from which to fabricate components are often specified. The logic in simply picking the best material follows only if the material ultimately meets the intended specification requirements and if the filter assembly manufacturer understand which material properties are governing.
Pall Corporation carefully evaluates all raw materials used to fabricate semiconductor gas filter assemblies. There are many important characteristics of 316L stainless steel that must be considered in designing these high purity assemblies. Type 316L stainless steel is a well characterized and understood alloy. Its good corrosion resistance is a result of both careful formulation of the major alloying elements (Fe, Ni, Cr, Mn, and Mo), as well as the control of critical trace constituents (C, S, and Si). Similar to other finished components, filter assemblies utilize stainless steel which has been further worked, i.e., rolled, drawn, machined, or welded.
In these cases, it is not sufficient to merely specify material chemistry. Each intended use of the stainless steel may entail slight modification of the basic specification, but the most commonly specified requirements resulting in a fine, electropolished surface finish are:
- balanced 316L chemistry.
- homogeneous austenitic microstructure.
- very few inclusions.
- fine grain structure.
Balanced chemistry is achieved by utilizing material which meets the requirements outlined in an appropriate existing specification. For example, SA2401 and SA4792 clearly define the chemical composition limits, as well as other physical properties, for 316L sheet and bar material, respectively (reference column 1 of Tables 1 and 2). The last three requirements are achieved by controlling the final melt of the stainless steel, and subsequent processing into the desired shape.3,4,5
Table 1: Materials Comparison: Stainless Steel Sheet or Strip
HEAT ID#
SPEC.--» |
N/A
SA240 |
848004
Pall Typical |
N/A
VAR Spec.Typ.1 |
ELEMENTAL(%): |
|
|
|
Fe |
BAL. |
BAL. |
BAL. |
Cr |
16-18 |
16.1 |
16-18 |
Ni |
10-14 |
10.0 |
10-14 |
Mo |
2-3 |
2.1 |
2-3 |
Mn |
2* |
1.8 |
<2 |
Si |
0.75* |
0.5 |
0.5 |
C |
0.03* |
0.019 |
<0.03 |
S |
0.03* |
0.0010 |
≤0.005 |
* = Maximum
Additional Criteria
GRAIN SIZE |
— |
6-7 |
Not Specified2 |
INCLUSION TYPE
(ASTM E-45) |
— |
NUMBER |
NUMBER |
Sulfides |
— |
0 |
1.53 |
Alumina |
— |
0 |
1.53 |
Silicates |
— |
0 |
1.53 |
Oxides |
— |
1.5 |
1.53 |
316L VAR does not specify (or result in) chemical composition which is of higher performance than SA240 with the noted exception of reduced sulfur.
2Typical measured values were within a window of 5-10.
3Not specified. Typical indicated.
Table 2: Materials Comparison: Stainless Steal Bar
HEAT ID#
SPEC.--» |
N/A
SA479 |
35439
Pall Typical |
N/A
VAR Spec.Typ.1 |
ELEMENTAL(%): |
|
|
|
Fe |
BAL. |
BAL. |
BAL. |
Cr |
16-18 |
17.1 |
16-18 |
Ni |
10-14 |
12.0 |
10-14 |
Mo |
2-3 |
2.1 |
2-3 |
Mn |
2* |
1.7 |
<2 |
Si |
1.0* |
0.45 |
≈0.5 |
C |
0.03* |
0.020 |
<0.03 |
S |
0.03* |
0.0020 |
≤0.005 |
* = Maximum
Additional Criteria
|
— |
5 |
Not Specified |
Type
(ASTM E-45) |
— |
No.: |
No.: |
Sulfides |
— |
0.5 |
2.03 |
Alumina |
— |
0 |
03 |
Silicates |
— |
0 |
2.53 |
Oxides |
— |
1.5 |
1.53 |
1 316L VAR does not specify (or result in) chemical composition which is of higher performance than SA479 with the noted exception of reduced sulfur.
2 Typical measured values were within a window of 5-10.
3 Not specified. Typical indicated.
In order to remove microscopic voids in the melt, as well as to further purify the metal by reducing certain contaminant impurities, many high purity stainless steels undergo a secondary melting process (e.g. vacuum arc remelt, known as VAR). This process reduces sulfides, silicates, phosphates, aluminas, and other impurities. Of these impurities, sulfur is the most detrimental to the achievement of fine surface finishe (assuming the other impurities are within normally specified limits).
These trace impurities tend to disturb (“break”) the stable austenitic structure and form inclusions which are less noble than the parent metal. When the inclusions are infrequent and very small in size, this may not be a problem. However, when very fine surfaces (<10 microinch Ra) are desired, all inclusions become apparent after final mechanical polishing or electropolishing. It is for this reason that 100X microscopic inspection after fine mechanical polishing is commonly used to quantify the inclusion content. Electropolishing often accentuates the defects in the surface by removing the less noble inclusions first, via preferential dissolution.
Grain structure must also be considered when choosing high purity stainless steel. Fine grained materials are preferable since they tend to be more homogeneous. As grains grow (e.g during slow cooling), there is more time for the austenite breakers to precipitate at the grain boundaries. This has the effect of transforming a clean dislocation of the structure (“healthy” grain boundary) into a site for inclusions. In addition, fine grained materials conduct electric current more uniformly. Uniform conductance is an important characteristic during the electropolishing process.
Stainless Steel Sheet Stock
All of the previously discussed characteristics have effects on the actual performance of the stainless steel. However, no one characteristic (chemistry or processing) will determine the efficacy of a stainless steel for use in a high purity gas system component. Table 1 provides a comparison of a typical high purity 316L stainless steel sheet stock material, as specified by Pall Corporation, to the SA240 specification and to the typical characteristics of VAR material.
Examination of the data reveal that the chemistry, grain size, and inclusion content are virtually identical. Figures 1a and b are photomicrographs of the Pall material vs. VAR material as they would be seen for inclusion enumeration. Both samples are very similar in appearance. Figures 2a and b show the respective grain sizes of the same samples. It should be noted that neither material shows significant dark spots (pitting) at the grain boundaries. Electropolishing of these apparently similar materials allowed for additional comparison. Sheet samples were initially mechanically polished to a fine finish, approximately 1 µm Ra ( 39 µ inch Ra), followed by a 30 second electropolish. Figures 3a and b illustrate the sheet stock materials after the electropolish, revealing similar surface appearance. Furthermore, these fine finishes were not measurably degraded in Ra by the electropolish process, as summarized in Figure 4.
Figure 4: Effect of Electropolish Process on Surface finish 316L Sheet Material
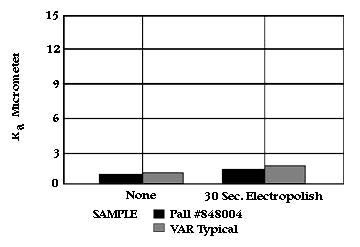
Stainless Steel Bar Stock
An identical evaluation was performed on bar stock samples. Table 2 summarizes the chemistry, grain size, and inclusion content for Pall specified bar stock, material conforming to SA479, and for a sample of material identified as VAR. Although both the Pall specified and VAR materials both met the basic chemistry criteria as specified in SA479, differences were observed. While both materials had sulfur contents below that specified by SA479, the Pall specified material was exceptionally low in sulfur content.
The grain sizes and inclusions for these materials are compared in Figures 5 and 6. The Pall material exhibited tight grain, and few inclusions. The VAR material was relatively large grained, and had a significant inclusion (pits and tears) content. Electropolishing of these samples under identical conditions resulted in a large differentiation between the materials. As seen in Figures 7a and b, the Pall specified material exhibited little pitting, while the VAR material exhibited severe pitting, indicative of the higher inclusion content and poorly controlled grain size of this VAR material. In addition, the VAR material experienced a notable degradation of the Ra value as a result of the electropolishing process. This material is clearly unacceptable for incorporation into a high purity gas component (Figure 8).
Effects Of Chemistry On Performance
The previously presented data on sheet and bar stock materials demonstrated that relative chemistries did effect electropolish results. Lower sulfur content resulted in better surface morphology. However, as discussed, individual chemistries will not necessarily predict material performance. For instance, Figure 9 illustrates a material which has severe inclusions even though it exhibited a sulfur content of less than 0.005%. It is therefore necessary to specify a material based on its actual performance as opposed to its chemistry alone.
Conclusion
The 316L stainless steel utilized in high purity semiconductor gas filter assemblies must be chosen carefully. The sheet and bar stock raw materials used must not only be specified for proper chemistries, but also for grain size
and inclusions. The suitability of the material to be formed, mechanically polished, and electropolished must also be evaluated. Since the fabrication of a complex assembly is more involved than, for instance, the drawing of high purity stainless steel tubing, each specification must take into account subtle differences in materials characteristics. The use of common specifications must be avoided unless the actual performance of selected materials has been fully evaluated. It is not sufficient to simply specify VAR and achieve fine surface finishes of optimal quality.
The data presented here demonstrated that materials of similar composition can perform very differently. It is for this reason that Pall Corporation has clearly specified materials whose performance exceeds that of standard materials and maintains a careful selection program . The proper selection of raw materials coupled with refined manufacturing techniques, will yield filter assemblies which are suitable for the rigorous demands of high purity semiconductor gas systems.
Photomicrographs 1a and 1b: Pall Material Versus VAR Material Showing Inclusions
Figure 1a. Inclusions: Pall 316L Sheet Material HT#848004
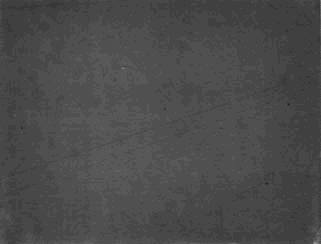
|
Figure 1b. Inclusions: 316L VAR Sheet, Typical
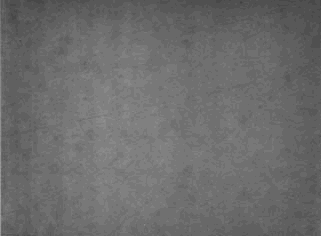
|
Photomicrographs 2a and 2b: Pall Material Versus VAR Material Showing Grain Size
Figure 2a. Grain Size: Pall 316L Sheet HT#848004 |
Figure 2b. Grain Size: 316L VAR Sheet, Typical |
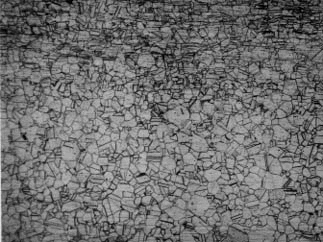 |
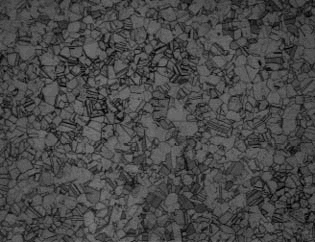 |
Photomicrographs 3a and 3b: Sheet Stock Materials After Electropolish
Figure 3a. After Electropolish: Pall Sheet Material HT#848004 |
Figure 3b. After Electropolish: 316L VAR Sheet, Typical |
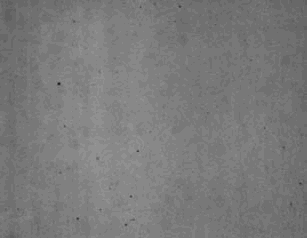 |
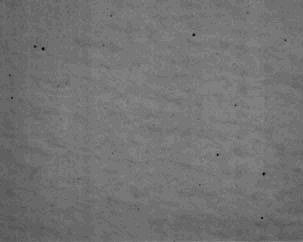 |
Photomicrographs 5a and 5b: Pall Material Versus VAR Material Comparison of Inclusions
Figure 5a. Inclusions: Pall 316L Bar Material HT#35439 |
Figure 5b. Inclusions: 316L VAR Bar, Typical |
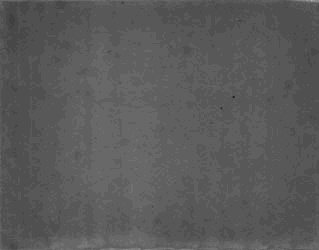 |
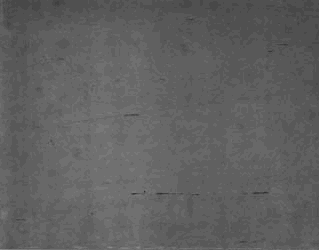 |
Photomicrographs 6a and 6b: Pall Material Versus VAR Material, Comparison of Grain Size
Figure 6a. Grain Size: Pall 316L Bar Material HT#35439 |
Figure 6b. Grain Size: 316L VAR Bar, Typical |
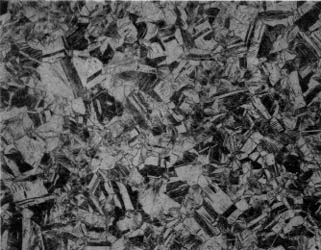 |
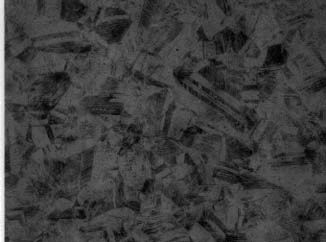 |
Photomicrographs 7a and 7b: Pall Material Versus VAR Material, Comparison After Electropolish
Figure 7a. After Electropolish: Pall 316L Bar HT#35439 |
Figure 7b. After Electropolish: 316L VAR Bar, Typical |
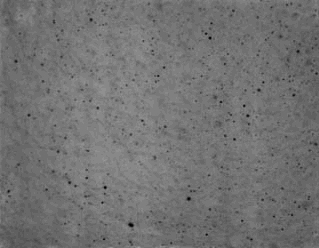 |
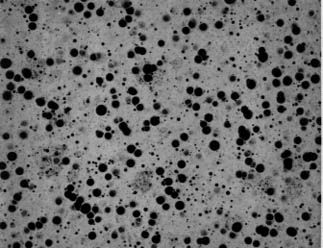 |
Figure 8: Effect of Electropolish Process on Surface Finish 316L Bar Material
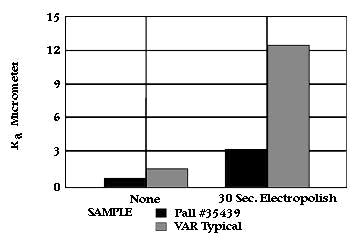
Photomicrograph 9: Material Showing Severe Inclusions
Figure 9. Low Sulphur 316L Sheet with Severe Inclusions
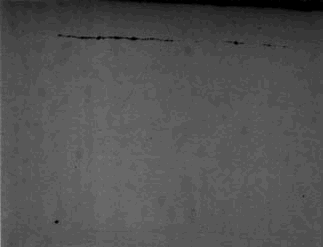
|
|
Note: Photomicrographs shown at 100X magnification.
References
1. ASME SA-240, “Specifications for Heat-Resisting Chromium and Chromium-Nickel Stainless Steel Plate, Sheet, and Strip for Pressure Vessels.” ASME BPVC Section II Part A (1989).
2. ASME SA-479, “Specifications for Stainless and Heat-Resisting Steel Bars and Shapes for Use in Boilers and Other Pressure Vessels.” ASME BPVC Section II Part A (1989).
3. ASM International Handbook Committee, METALS HANDBOOK 10th Edition, Vol. 1, “Properties and Selection: Irons, Steels, and High Performance Alloys.” ASM International, Materials Park, OH (1990).
4. A. J. Sedricks. “Corrosion of Stainless Steels.” John Wiley and Sons, NY (1979).
5. M. G. Fontana and Norbert D. Greene. “Corrosion Engineering,” 2nd Edition. McGraw-Hill, NY (1978).
Related References:
Selection 316L Stainless Steel for High Purity Semiconductor Gas Filter Assemblies
Comparison of Grades 316 and 316L to 316Ti
|